Ampere’s law states that the integral of magnetic field density (B) along an imaginary path is equal to the product of the permeability of the free space and the current enclosed by the path.
Ampere’s Law Equation
Ampere’s law is a mathematical statement that describes the link between currents and the magnetic fields they produce. It is therefore the magnetic counterpart of Gauss’ law, which describes the relationship between charges and their electric fields.
Ampere’s Law allows us to calculate (analytically) the magnetic field produced by an electric current in configurations with a high degree of symmetry.
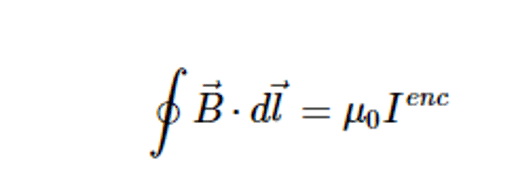
The integral on the left is known as a “path integral.” The circle symbol on the integral indicates that it is an integral over a “closed” path; that is, a path with the same beginning and ending locations. Ienc is the net current that crosses the surface specified by the closed route, often known as the path’s “current contained.” μo is the permeability of free space, which is a constant that’s always equal to 1.257 x 10^-6.
In some literature, The mathematical statement of Ampere’s Law is also given as:
∮B·ds = μ0 * I
where:
- ∮B·ds is the line integral of the magnetic field B along a closed loop or path
- μ0 is the permeability of free space, a constant value equal to 4π × 10^-7 N/A^2
- I is the net current passing through the surface enclosed by the loop.
In simple words, Ampere’s Law relates the magnetic field created by a current-carrying wire to the current passing through it. The integral on the left-hand side of the equation represents the magnetic field integrated over a closed loop, while the right-hand side represents the total current passing through that loop.
Applications of Amper’s law
Some of the applications of Ampere’s law are given below:
- This law gives us a way to calculate the magnetic field that is produced as a result of an electric current moving through a wire of any shape
- The primary use is, of course, computing the magnetic field produced by an electric current. This is helpful in electromagnets, motors, generators, transformations, and other applications.
Features of Ampere’s Law | Description |
---|---|
Definition | A fundamental law of electromagnetism that relates the current flowing through a closed loop to the magnetic field created by that current. |
Significance | Ampere’s Law provides a mathematical relationship between the current and the magnetic field, which is crucial for understanding the behavior of magnetic materials and designing electromechanical devices. |
Symmetry | The law has a high degree of symmetry, which means that the magnetic field generated by a current-carrying wire is the same at every point along a circular path around the wire. |
Applications | Ampere’s Law is used in a wide range of applications, including in the design of electric motors, transformers, and generators. It also has several daily life examples such as: |
Daily Life Examples | Magnetic locks on doors, which use the principle of Ampere’s Law to secure the door using a magnetic field. Hairdryers, which use a motor that operates on the principles of Ampere’s Law to turn the fan blades and produce hot air. MRI machines in medicine, which use powerful magnetic fields to create detailed images of the inside of the body. | |
Multiple Choice Questions
- Which of the following is a correct statement about Ampere’s Law?
A) It is only applicable to a straight current-carrying wire.
B) It is only applicable to a closed loop.
C) It relates the magnetic field around a closed loop to the current passing through the loop.
D) It relates the electric field around a closed loop to the current passing through the loop.
Answer: C) It relates the magnetic field around a closed loop to the current passing through the loop.
- Which of the following is a correct expression of Ampere’s Law in differential form?
A) ∇ × B = μ0J
B) ∇ · B = μ0ρ
C) ∇ × E = -∂B/∂t
D) ∇ · E = ρ/ε0
Answer: A) ∇ × B = μ0J, where J is the current density.
- Which of the following statements is true according to Ampere’s Law?
A) The magnetic field inside a current-carrying wire is always zero.
B) The magnetic field inside a current-carrying wire is always non-zero.
C) The magnetic field inside a current-carrying wire is proportional to the current.
D) The magnetic field inside a current-carrying wire is inversely proportional to the distance from the wire.
Answer: A) The magnetic field inside a current-carrying wire is always zero. This is a consequence of Ampere’s Law when applied to a long, straight, current-carrying wire.
- Ampere’s Law is a consequence of which fundamental law of nature?
A) Law of Conservation of Energy
B) Law of Conservation of Momentum
C) Maxwell’s Equations
D) Newton’s Laws of Motion
Answer: C) Maxwell’s Equations. Ampere’s Law is one of four equations known as Maxwell’s Equations, which describe the behavior of electric and magnetic fields in space and time.
Solved Numerical Problems
Practice Exam Questions
- A long, straight wire carries a current of 5 A. What is the magnitude of the magnetic field 2 cm away from the wire?
Solution: Ampere’s Law states that the line integral of the magnetic field around a closed loop is equal to μ0 times the current passing through the loop. In this case, we can assume a circular loop around the wire with a radius of 2 cm. The circumference of the loop is 2πr = 4π cm. Using Ampere’s Law, we get:
∮B·ds = μ0 * I
B * 2πr = μ0 * I
B = (μ0 * I) / (2πr)
B = (4π × 10^-7 T·m/A) * (5 A) / (2π × 0.02 m)
B ≈ 1.0 × 10^-4 T
- A wire loop with a radius of 10 cm carries a current of 2 A. What is the magnitude of the magnetic field at the center of the loop? Hint: Use Ampere’s Law and assume a circular loop around the wire.
Solution: Ampere’s Law states that the line integral of the magnetic field around a closed loop is equal to μ0 times the current passing through the loop. In this case, we can assume a circular loop around the wire with a radius of 10 cm. Since the current is constant around the loop, we can use symmetry to argue that the magnetic field is constant at the center of the loop. Using Ampere’s Law, we get:
∮B·ds = μ0 * I
B * 2πr = μ0 * I
B = (μ0 * I) / (2πr)
B = (4π × 10^-7 T·m/A) * (2 A) / (2π × 0.1 m)
B ≈ 6.3 × 10^-6 T
Biot Savart Law
The Biot Savart Law is a mathematical expression that defines how a continuous electric current creates a magnetic field. It relates the magnetic field to the amplitude, direction, length, and closeness of the electric current. Ampere‘s circuital law and Gauss’ theorem are both compatible with the Biot–Savart law. The Biot-Savart law, which functions similarly to Coulomb’s law, is critical in magnetostatics.
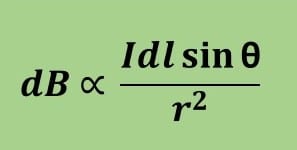
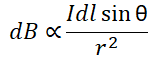
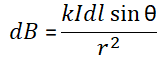

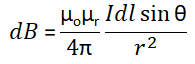
Where k is a constant that is determined by the magnetic characteristics of the medium and the system of units used.
Related Topics
Electric potential difference and Ohm’s law
Power Units- The Basics
Conductor And Insulator
Malus Law- Definition, Concept, and Examples
Electric Field Units & Definition
The energy of Light| Uses of Light Energy
Summary
- Ampere’s Law relates the magnetic field around a closed loop to the current passing through the loop.
- The law can be used to calculate the magnetic field around a current-carrying wire or any other current distribution with cylindrical symmetry.
- The law states that the magnetic field is proportional to the current passing through the loop and inversely proportional to the distance from the wire.
- Ampere’s Law is a fundamental law of electromagnetism that plays a key role in designing electric motors, generators, and transformers.
Frequently Asked Questions
1. What is the magnetic permeability
Magnetic permeability is defined as the magnetic induction to magnetic intensity ratio. It is a scalar quantity that is denoted by the symbol. Magnetic permeability is a measure of a material’s resistance to a magnetic field or the amount to which a magnetic field may pass through it. It is measured in Henries per meter (H/m), which is equivalent to newtons per ampere square.
Magnetic permeability is denoted as μ and can be expressed as μ = B/H, where, B is the magnetic flux density which is a measure of the real magnetic field within a material and is defined as a concentration of magnetic field lines of magnetic flux per unit cross-sectional area.
H stands for magnetic field strength, which is a measurement of the magnetizing field created by electric current flowing through a wire or coil.
2. What is ampere’s law?
According to Ampere’s law, the integral of magnetic field density (B) along an imaginary line is equal to the product of free space permeability and current enclosed by the path.
Conclusion
In conclusion, Ampere’s Law is a fundamental concept in electromagnetism that allows us to understand and calculate the magnetic fields produced by current-carrying wires and other cylindrical current distributions. Its practical applications are vast and can be found in numerous fields, including electrical engineering, physics, and telecommunications, leading to advancements in technology.
More Links
- BCl3 Lewis Structure in four simple steps - November 1, 2023
- PH3 Lewis Structure in four simple steps - October 8, 2023
- PF3 Lewis structure in four simple steps - September 24, 2023